HOME > Research Summaries > Electrochemistry-Based Bionic Devices
Research Summaries
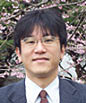
Electrochemistry-Based Bionic Devices
Matsuhiko Nishizawa
Professor
Department of Bioengineering and Robotics, Graduate School of Engineering
E-mail:
1. Introduction
The interfacing between the biomolecules and device materials is one of the most important subjects for both in-vitro and in-vivo medical devices. We have been studying the potential use of microelectrode techniques as the tool for controlling bionic interfaces, and developed an electrochemical methods to control cell adhesion and growth. The technique is simple enough to be integrated to the small and closed systems such as microfluidic devices. In addition to the micropatterning of proteins and cells, the in-situ microcircuit formation with conducting polymers has been studied as a potential interface to cellular networks.
The biological fuel cell is another electrochemical bionic device we are studying. The energy conversion efficiency of the living system is very high, and the reconstruction of the protein-based bioenergetic system in vitro is a challenge to develop bio-adaptive fuel cells.
The followings are the research topics that we will study during the GCOE program.
1) Enzymatic Bio- Fuel Cell
2) On-Demand Immobilization Biochip
3) Cellular Network Chip
4) Conducting Polymer-Based Cellular Electronics
2. Bionic Fuel Cell
Electric power derived from dispersed ambient energy has attracted attention as ubiquitous portable power. A potential option of portable power source is biological fuel cell that use enzyme as an electrocatalyst to generate electricity from such biological fuels as alcohols and carbohydrates. The high reaction selectivity of enzymes would allow separator-free design and power generation from complex natural fuel solutions without purifications, that is, direct utilization of refreshments containing sugar, plant saps, and biological fluids such as blood.
The enzymatic biofuel cells would mostly stand out in biomedical applications, especially for the power generation directly from biofluids, tissue fluids and blood, containing glucose (ca. 5 mM), lactate (ca. 1 mM) and oxygen (0.1 mM in arterial blood).
We have studied the enzyme anode for glucose oxidation composed of a bi-layer polymer membrane, the inner layer containing diaphorase (Dp) and the outer, glucose dehydrogenase (GDH). The Dp membrane was formed from a newly synthesized Vitamin K3-based mediator polymer. The enzyme cathode for oxygen reduction can be prepared by using bilirubin oxidase (BOD). The enzyme biofuel cell generates power comparable to a button battery (~0.2 mW) in bovine serum.
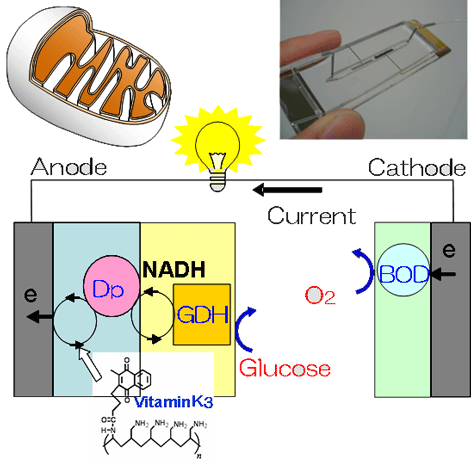
Fig. 1. Schematic illustration showing structure of the enzyme-based Biological Fuel Cell.
3. Electrochemical Biolithography
The controlled immobilization of bio-elements (proteins and cells) onto substrate surfaces is one of the most important subjects for advanced biotechnology. We have developed a technique named “Electrochemical Bio-lithography”, which enables micropatterning of biomaterials even within a micro-fluidic channel. The technique is based on our recent finding that the biophobic nature of heparin-coated substrates rapidly switched to a protein- and cell-adhesive one when exposed to an oxidizing agent such as hypobromous acid, which can be produced by the electrochemical oxidation of bromide ion in aqueous solution. The electrochemical bio-lithography is a remarkably simple technique requiring only some microelectrode and a voltage source (1.7 V, DC), and thus its integration to the conventional microfluidic chip has been easily achieved.
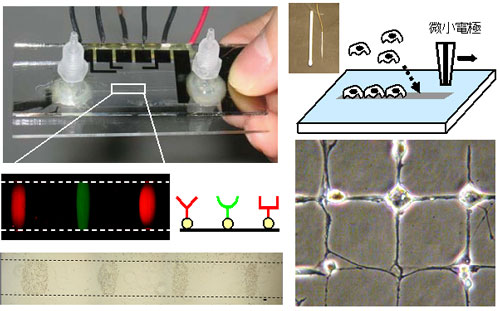
Fig. 2. The newly developed surface technique “Electrochemical Biolithography” can immobilize proteins
and living cells, even in the pre-assembled microchannel, and even during cell cultivation.
4. Cellular Network
Another important advantage of the electrochemical Biolithography is its mildness which enables the multi-micropatterning with different kinds of cells as to give hetero-culture networks. Furthermore, the navigation of cellular growth and migration has been succeeded, since the generated oxidizing agent can selectively denature only the surface coating of albumin and heparin nearby the cells without a significant damage on the pre-existing cells. These unprecedented features can be integrated to fluidic devices, and would expand possibility of micro-TAS systems for the cellular diagnosis, drug screening and cellular engineering.
5. Conducting Polymer-Based Cellular Electronics
Engineering the interfacial contact between microelectrodes and biological cells is of central importance to the advancement of cell-based bioelectronic devices. Conducting polymers such as polypyrrole (PPy) and poly (3,4-ethylenedioxythiophene) (PEDOT) are excellent candidates for bioelectrode materials owing to their inherent electrical conductivity, controllability of surface biochemical properties, and biocompatibility. In particular, their large surface area due to a fibrous structure increases the interfacial electrical capacity, ensuring noninvasive electrical stimulation of biological cells. On the other hand, recent technical progress in cellular micropatterning has made bioassays possible using cellular networks combined with electrode arrays. Such advanced in-vitro cellular assays also require a high-capacity noninvasive electrode.
We have developed a technique to induce the anisotropic lateral growth of PPy along the insulating glass substrate. The PPy film laterally grown around the electrode anchors the whole film and significantly enhances film adhesion. The anchored PPy film was stable even during a long-term cultivation, and serves as an electrode for reproducible, noninvasive, external stimulation of a cultured excitatory cells. This anisotropic polymer growth will be applied to the formation of hybrid micropatterns of conducting polymer and living cells on a chip.
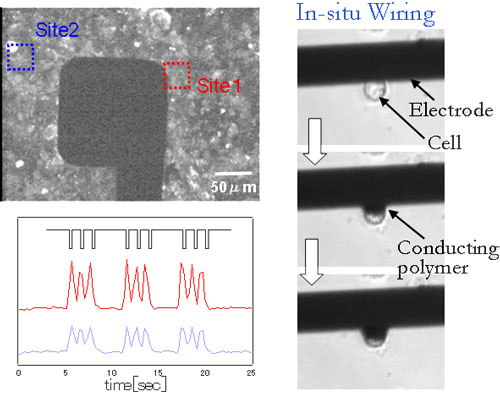
Fig. 3. A sheet of cardiac myocyte was noninvasively stimulated by a PPy-coated electrode.
The lateral growth of PPy was induced to make electrical contact with living cells.
References
[1] Bionic Fuel Cells:
Electrochem Commun 7, 643, 2005. Electrochim Acta 52, 4669, 2007.
[2] Electrochemical Biolithography:
Langmuir 20, 16, 2004. J Am Chem Soc 126, 15026, 2004. Langmuir 21, 6966, 2005. Anal Chem 78, 5469, 2006.
[3] Cellular Network:
Langmuir 18, 3645, 2002. Biomaterials 24, 4239, 2003. Lab Chip 3, 313, 2003. JSME Int J Ser C 47, 956, 2004. Langmuir 22, 10784, 2006.
[4] Conducting Polymers:
Electrochim Acta 44, 3629, 1999. Biomaterials 28, 1480, 2007. Langmuir, 2007 (in press).